We all know that temperatures are increasing, and scientific evidence shows that this is directly linked to anthropogenic emissions of greenhouse gasses; the question is no longer ‘What is happening? and why?’ but ‘What can we do about it? And, how quickly?’
The high-level answer was provided by the UN Framework Convention on Climate Change (UNFCCC) and later ratified by 195 nations in the signing of the Paris Agreement in 2015; to hold the increase in global average temperatures to well below 2°C above pre-industrial levels and to pursue efforts to limit this temperature rise to 1.5°C.
Climate science is clear that the eventual extent of global warming is proportional to the total amount of greenhouse gasses (GHGs) that human activities add to the atmosphere; to stop the rapid rise in temperatures we must reduce GHG emissions… the longer it takes us to do so, the more the climate will change. So, what can we change to reduce GHG emissions from human activities?
Well, the built environment is estimated to contribute about 40% of total global greenhouse emissions, this simultaneously represents a threat to achieving the goals of the Paris Agreement and an opportunity for businesses like ours to become a part of the solution.
An electric product such as a Heat Pump will have a significantly lower WLC carbon than an alternative such as a Gas Boiler
The context and the built environment
When talking about the emissions generated from the built environment, it is important to understand what we mean when we refer to embodied carbon, operational carbon, or whole-life carbon.
Embodied Carbon is the total GHG emissions generated to produce a built asset. This means that to calculate the embodied carbon for a building we need to understand the environmental cost to the planet for the extraction, processing, manufacture, delivery and assembly of every single product or material used in its construction.
Throughout a building’s lifetime some maintenance or replacement of these products or materials will be necessary – and this also needs to be measured as a part of calculating embodied carbon.
At the end of the building’s useful life more emissions will be produced because the asset needs to be deconstructed, and the products within disposed of, and this also needs to form part of an embodied carbon calculation.
Operational Carbon
You have probably noticed something missing from the above – the emissions generated by a building during its useful life – this is what we call Operational Carbon.
Operational Carbon refers to the total GHG emissions produced by a building during its useful (operational) life. These emissions arise from energy consuming activities like the heating, cooling, ventilation, and lighting needs of the building – also known as ‘regulated’ emissions as they fall under Part L of the Building Regulations ‘Conservation of fuel and power’ – as well as other ‘unregulated’ emissions such as those from appliance use and small power plug loads from the day-to-day activities of the people using it.
Before we move on to Whole Life Cycle carbon, it is important to make the distinction between direct and indirect emissions.
Direct emissions are those generated from sources that are owned or controlled by the reporting entity. In the case of the built environment this means all emissions generated on site, a good example of this would be the burning of natural gas for the provision of heat; gas is pumped into the building, burned as a fuel source and releases GHGs directly into the atmosphere as a result.
Indirect emissions are those generated because of the activities of the reporting entity but that occur at a location owned or controlled by another entity.
A good example of this would be the emissions generated from the electricity used in the building; these emissions are not produced on the site of the building itself but are an indirect result of the building’s electricity demand.
This is an important distinction as the emissions generated from burning fossil fuels is fixed - each unit of gas burned releases the same emissions as the last – while the indirect emissions from electricity use depend on the carbon intensity of the methods used to generate that electricity – which can change.
As we replace fossil fuels with renewables on the journey to decarbonise our electricity generation mix here in the UK, the indirect emissions resulting from this energy use will decrease over time. This is where the argument for electrification comes from; each time we can substitute direct emissions for indirect emissions we can realise a marginal reduction in operational carbon every year.
Whole Life Cycle (WLC) Carbon: choosing the right measurement of success.
This is where understanding the Whole-Life Cycle (WLC) carbon of a building becomes important – considering both the embodied carbon and operational carbon of a building allows us to predict the total GHG emissions generated throughout the building’s entire life.
Using WLC carbon as a measure also means we can get a better understanding of the trade-offs that can be made between embodied and operational carbon, and their cumulative impact over time.
To illustrate this, we can consider how the decision-making process of a designer regarding insulation may change depending on whether they want to consider embodied, operational or WLC carbon of their building.
The designer looking to reduce the embodied carbon of their building may opt for a lower level of insulation, reducing the carbon emissions associated with its manufacture, transportation, and eventual disposal but increasing the future operational carbon of the building through a loss in energy efficiency.
The designer looking to reduce the operational carbon of their building may instead want to maximise the level of insulation, reducing the operational carbon of the building through increased efficiencies from lower levels of heat loss, but increasing the embodied carbon of the building by the amount of embodied carbon emissions from this additional insulation.
Each of these examples presents an alignment problem – assessing embodied or operational carbon in isolation can have unintended consequences.
By contrast, a designer who considers the WLC carbon of their building will consider the relative trade-offs between embodied and operational carbon in the context of their cumulative impact over time and select the path that will minimise the total carbon cost of that building when it comes to the end of its useful life.
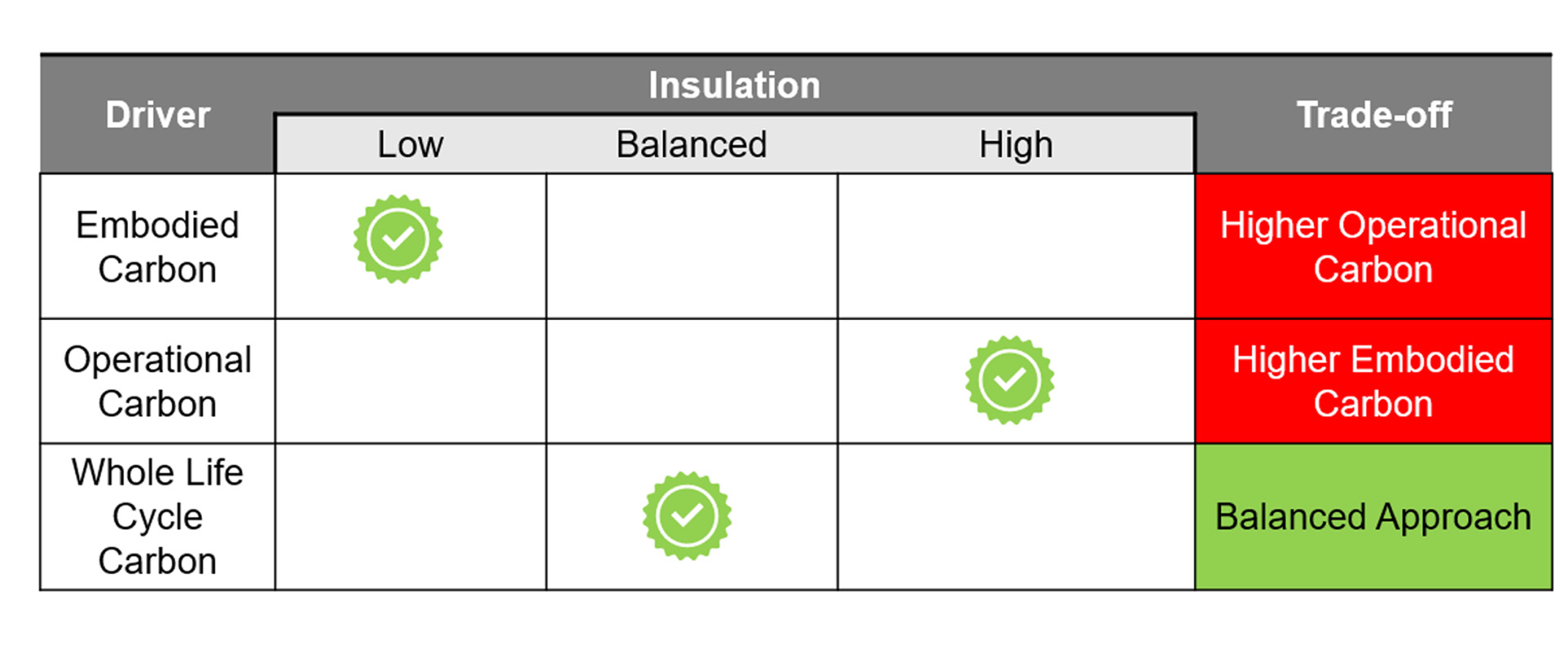
A trade off
We need to find a balanced approach
Our products and the Decarbonisation of Heat
This sets the scene for us to look at some of the other ways that Mitsubishi Electric products can be deployed to reduce the WLC carbon of a building.
As the example above shows, you can make a trade-off between the embodied and operational carbon in a building by changing energy efficiency aspects such as insulation - the key is to look at this trade-off in the context of WLC to find a ‘goldilocks zone’; a balance between the resource intensity of the building’s construction and the efficiency of its operation over the long-run.
Another way that we can seek this balance is through the substitution of direct emissions for indirect emissions, AKA electrification.
As the UK government continues to move away from using fossil fuels for the generation of electricity towards a greater mix of renewables, the carbon cost of each unit of electricity will decrease.
Research from the Committee on Climate Chance (CCC) shows that direct GHG emissions from buildings can be shown to contribute 17% of the UK’s total emissions in 2019, with the lion’s share coming from the use of fossil fuels for heating and hot water. Approximately 74% of our demand for heating and hot water is met from natural gas, making this a vital area to transform to realise GHG emission reductions.
As I have already mentioned, each unit of gas burned will always produce the same amount of GHG emissions as a result, but each unit of electricity used has the potential to have lower associated emissions due to the cleaning energy grid.
This means that assuming the heating requirement remains constant, an electric product such as a Heat Pump will have a significantly lower WLC carbon than an alternative such as a Gas Boiler.
In fact, thanks some of the work we have been doing to provide our customers with the data they need to understand the embodied and operational carbon of our products we can demonstrate this difference in WLC carbon using the below graphs of a simple space heating solution deployed in a typical residence over 15 years.
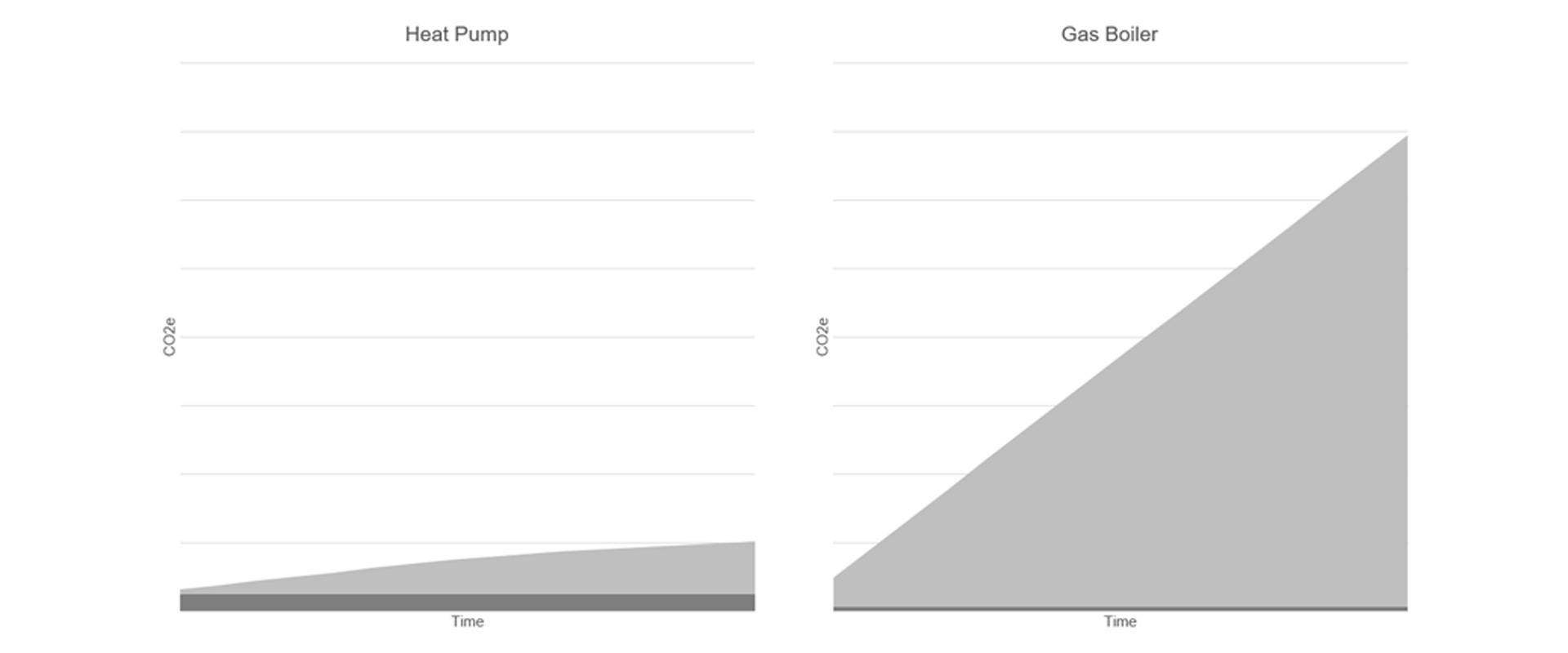
Heating
A heat pump versus a gas boiler - These calculations were conducted using some assumptions based on a typical air to water heat pump using ERP label energy data and efficiency as per the ERP fiche. The boiler calculation assumed a boiler efficiency of 97%, and used embodied carbon estimates based on the PEP Ecopassport and CIBSE’s TM65 calculation methodology
Higher embodied / Lower Operational
Using the TM65 Mid-Level Embodied Carbon Calculation methodology we can accurately measure the embodied carbon of a 5kW Heat Pump and for an equivalent Gas Boiler on the right – this is shown as the dark grey region on each of the graphs above.
As you can see, a Heat Pump has a significantly higher embodied carbon associated with it than does a Gas Boiler, and this can be attributed to the fact that it is a heavier product with more complex component parts which, in turn, have higher associated embodied carbon, and to the significant contribution that refrigerants make to an embodied carbon calculation.
This means that a designer considering each system in the context of only the dark grey embodied carbon would want to install the Gas Boiler system and then pat themselves on the back, happy with how ‘Sustainable’ they were being.
However, operational carbon is by far the more significant factor contributing to WLC carbon for both systems - as we can see from the light grey region on each of the graphs above. In fact, the difference in the operational carbon between these systems is so great that when taken in the context of WLC carbon we can show that the total CO2e of running the Heat Pump system on the left for 15 years is equivalent to running the Gas Boiler system on the right for less than 2 years.
In fact, the difference in operational carbon between these systems is so stark that you could replace your Heat Pump each year – effectively adding the embodied carbon of the product to the WLC carbon of the building 15 times, and you still would not reach the same level of WLC carbon of the Gas Boiler system.
Interestingly, while the relative significance of the embodied carbon element of each of these systems differs depending on their associated operational carbon – the embodied carbon element will contribute much more to the WLC carbon in the case of a Heat Pump than in the case of the Gas Boiler – the operational carbon is still the most significant factor for WLC carbon, regardless of which system we consider.
This is important, as we need to be focusing first on making improvements that will provide the greatest reductions in total GHG emissions over time – and as we can clearly see above, this is to reduce the operational carbon of our heating systems.
Key Takeaways
So, what should you take away from this article?
The key takeaway is that the construction industry in the UK is on a journey; the contribution made by the built environment is significant to the UK’s total GHG emissions and it must be reduced. The UK government has committed to a Net Zero carbon emissions target for 2050 and already we have seen this translate into changing drivers in the construction industry.
As a part of this shift manufacturers like us are being asked to provide ever more transparent levels of data relating to the embodied carbon of our products and we at Mitsubishi Electric have been working hard to provide this in the form of the CIBSE TM65 Embodied Carbon Calculations we have been releasing for our products.
Whilst we can use this data to provide insights and analysis that will help both ourselves and our customers contribute to achieving Net Zero 2050 we are still at the start of this journey.
The most important aspect of this is for us – and the industry as a whole – to make sure we get our data capture and tracking right, then to use this to continuously improve both our products, and the information that we can provide to our customers.
Jack Bain is a member of the Sustainability Team